Τitle
Mechanical response and FEM modeling of porous Al under static and dynamic loads
Mechanical response and FEM modeling of porous Al under static and dynamic loads
Advanced Engineering Materials, 16 (3), pp. 289-294.
Many researchers have turned to numerical simulation to gain insights into the relationship between foam microstructure and bulk properties.[1–5] However, simulating foam mechanical response can become a computational challenge for two major reasons. First, realistic foam microstructures are complex and difficult to characterize experimentally or based on approximations.[6] Even idealized foam microstructures are difficult to discretize with solid meshing algorithms, as required by the finite element method (FEM). Second, to simulate foam response over the range of deformations routinely utilized in engineering applications requires simulation into the densification stage. Hence, the material deformations of interest are large and can result in numerical inaccuracies as Lagrangian meshes become distorted or Eulerian meshes require extensive material advection. Furthermore, the transition from plateau to densification regimes is driven by contact of the collapsing foam microstructure and contact algorithms themselves are an area of evolving research. The proposed method addresses the first issue by substituting the real geometry with an equivalent one.
Until now, the foam geometry transition from real to computer world was realized by mainly applying X-ray computed tomography techniques.[7] On one hand, X-ray computed tomography (X-ray CTor X-ray micro CT) offers the advantage of the actual geometry reproduction of the scanned specimen, but at the cost of the necessary data post-processing, a time-consuming process which cannot guarantee a trouble-free geometrical model. On the other hand, initial CAD-based methods implement special-shaped cells, such as dodecahedron, icosahedron, tetrakaidecahedron, to model an equivalent geometry in terms of specific evaluation parameters such as foam porosity, pore ratio, mean pore size distribution, cell area, and circularity.[8,9] The obvious disadvantage is the absence of cell dimension randomness, which limits the functionality of these models. Voronoi tessellation is a technique that enables the division of multi-dimensional spaces into subspaces.[10–12] When applied to 3D space produces polyhedra with planar faces, having random edge sizes and number of faces.[13] Since size irregularity is a pivotal parameter for describing foam geometry, voronoi tessellation has been lately introduced by researchers.[14,15]
Laguerre–Voronoi tessellations are commonly used, up to now, for generating polyhedral, which are later transformed into open structures, mimicking the geometry of open-cell foams.[10–12] In the present paper, a different procedure is applied for converting generated polyhedra into closed-cell porous structures, considering also the ellipsoidal-like shape of the cells of the actual aluminum foam. In addition, the produced geometry was used for conducting FEA simulations employing DEFORM 3D software package, to examine the effect of strain rate on the structural response of the metal foam, which is subjected to high velocity impact. The representation of the complex geometry of metal foam, employing Non Uniform Rational B-Splines (NURBS), is proposed to render possible a fail-free volume discretization using finite elements. In this context, the closed-cell foam geometry was approximated combining a parametric 3D Voronoi cell volume partitioning with sophisticated CAD algorithms. The applicability of this method in the investigation of cellular materials mechanical response is investigated, while the developed FEM model in the actual sample size, and not in just a portion of it, is validated by static and impact tests.
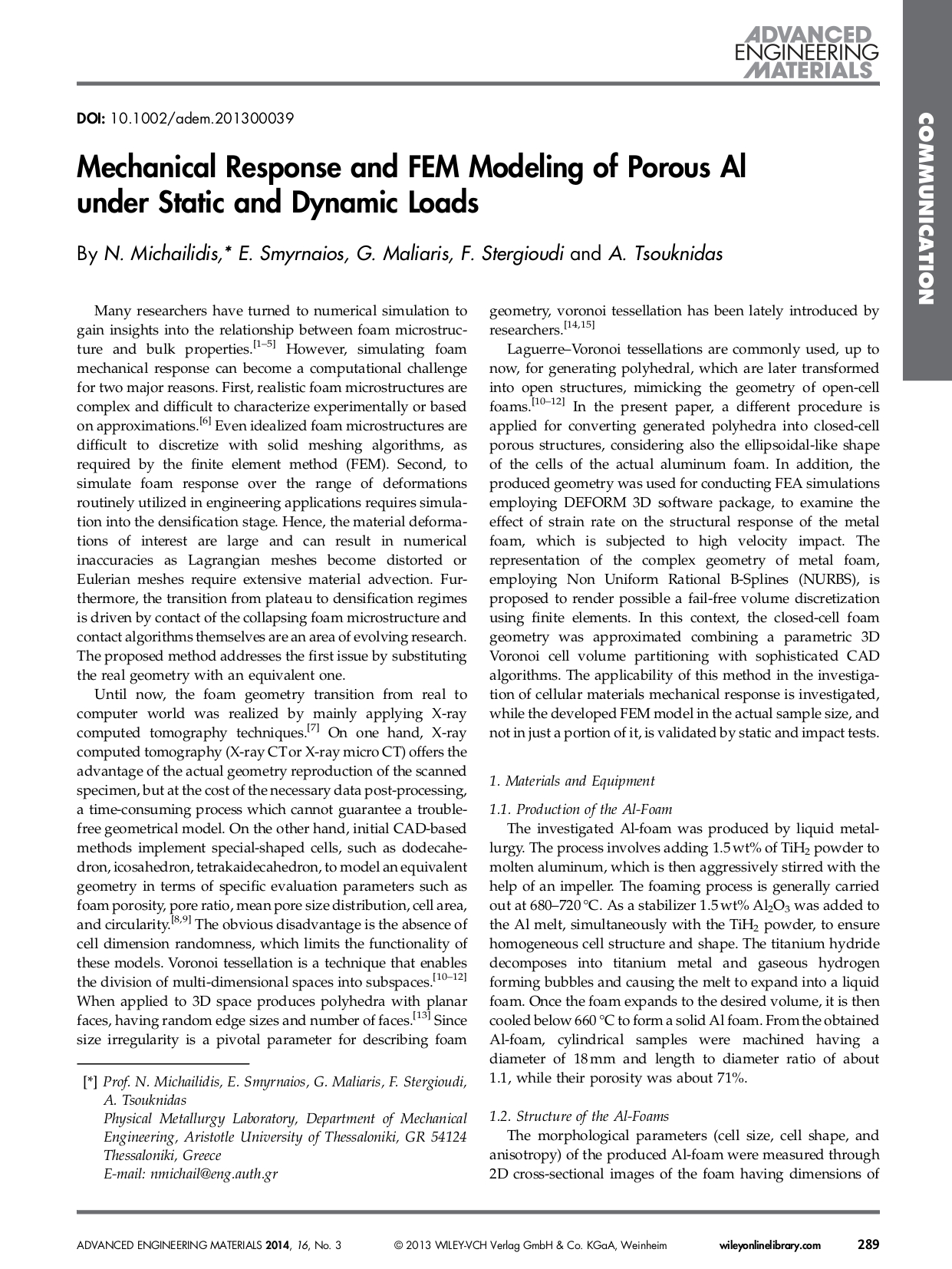